The Power of Silicon
Most rock-forming minerals are silicates, silicon-based chemical compounds. Like carbon holds the secret of living things, silicon has the power to rock!
Silicon is the most important element in the Earth’s crust and below. The crust is the outermost and rather thin layer of our planet. A few tens of kilometers below, the composition changes and we talk about a mantle that surrounds the Earth’s metallic core. Combined with oxygen (the most widespread element in the Earth's crust), silicon creates crystal structures that host metal and alkaline atoms to form the minerals known as silicates. A planet is made of rock. A rock is an assemblage of minerals. Chemical compounds tend to crystallize when they precipitate from a solution or when they solidify from a liquid (think of ice crystals on freezing water). The crystals that form have shapes that reflect the orientation of the atomic bonds in the molecule. That is why they tend to have precise geometrical shapes. We call them minerals and the most important rock-forming minerals are the silicates.
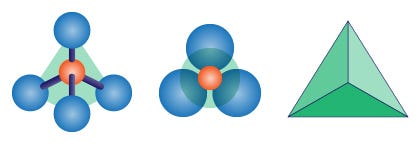
The silicon atom has 14 protons in its nucleus. The number of protons, known as the atomic number, dictates the properties of an element. That is, silicon behaves in a certain way because it has 14 protons in the nucleus. If they were 13 it would have been aluminum, an element with different properties. 15 protons would make it phosphorus. Electrons “kind of” orbitate far away from an atom’s nucleus. Usually they tend to balance an atom’s proton content: protons have a positive electrostatic charge while electrons, although much smaller than protons, have the same amount of electrostatic charge but of the opposite kind: they are negative. It happens that some elements have an equal number of electrons balancing out the charge of the protons in the nucleus. Helium, argon, neon are like this and we sarcastically call them “noble” gases because they don’t like to bond with any other elements - they are complete as they are. Mid-class elements, gold included, need the collaboration of others to complete the charge balancing: some lend electrons, some borrow them. Silicon needs to borrow 4 electrons in order to balance the 14 positive charges in its nucleus. More than often, silicon likes to borrow from oxygen, which has a couple of exceeding electrons to spare. Therefore, one silicon atom needs to borrow from a couple of oxygens. That’s why the most common compound containing silicon has the formula: SiO2. We call it “silica”. When silica forms crystals we often call it “quartz”.
Silicon is not the only important “central” element in rock-forming minerals. The guy with 13 protons, aluminum, is also well widespread. Bonding with oxygen it forms “alumina” Al2O3 or aluminum oxide, a more complicated molecule known as the mineral “corundum”. But silicon has the power. When more oxygen atoms are around, silicon tends to grab four for itself, one for each of its exceeding positive charges (that in chemistry are called valences). The result is a silicon atom surrounded by 4 oxygen atoms. Each oxygen has therefore a spare electron to share. The entire group will result in having a -4 charge. Since the 4 negative charges from the 4 oxygen atoms repel each other, they will tend to stay as far away as they can. The result is that they will be oriented at typical angles from each other: the negative charges around the SiO4 silica group will be oriented as the corners of a tetrahedron, the geometrical solid shape with 4 triangular faces. That’s why we geologist always talk about “silica tetrahedrons” when we reason about rock-forming minerals.
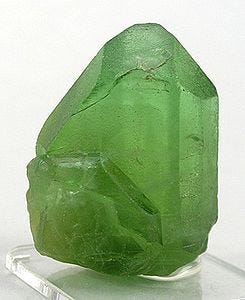
The simplest form of silicate has just one silica tetrahedron. They go by the name of “nesosilicates”. Such basic structure with 4 negative bonds available has the tendency to attract metals, that are usually desperately in need of electrons to borrow. The most common are iron (Fe) and magnesium (Mg). The single silica tetrahedron can bond both kinds of atoms (2 of each) in a sort of solid mixture containing different proportions of iron and magnesium: (Fe,Mg)2SiO4. We call this kind of minerals “olivines” or “peridots”.
As a matter of fact, we could oversimplify by saying that most rocks are made of iron and magnesium silicates. But especially when the silica tetrahedrons bond to each other to form chains, more electrons are available and different atoms also join the party. The so called “alkali” elements such as calcium, sodium and potassium (Ca, Na and K) are the most important ones. More complicated silicates can be a combination of silica tetrahedron structures hosting a mix of metals and alkali.
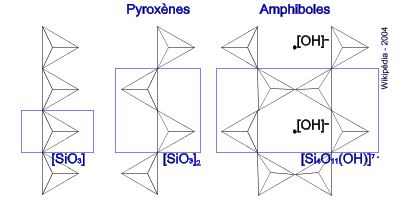
The power of silicon is that silica tetrahedrons can polymerize in a way rather similar to that of carbon in organic matter. Carbon bonds more easily with hydrogen and the basic compound, methane, has a similar structure to silica, with a central carbon surrounded by 4 hydrogens. The ability of carbon is to polymerize in chains so long that they can store huge informations based on where in the chains certain atoms or molecular groups are attached. That is how the code of life is generated. Silicon can’t make such long polymers but the behavior is fairly similar. Silica tetrahedrons can share oxygen atoms creating chains to form the minerals we call “inosilicates” or chained silicates. Such chains can be arranged in more complicated ways forming 2-D and 3-D structures. Different structures give rise to different types of silicate minerals. Single-chain inosilicates are called “pyroxenes”, while double-chain inosilicates are called “amphiboles”. More complicated 3-D structures are those of “tectosilicates” or frame silicates. They make up almost 75% of the Earth’s crust. Some tectosilicates called “feldspars” contain exclusively alkali elements (and also some aluminum in the tetrahedrons). The feldspars containing a mix of sodium and calcium are called “plagioclase”. Often, the ratio of calcium and sodium in a plagioclase determines the type of rock.
Aluminium can replace some of the silicon atoms at the center of tetrahedrons like in the tectosilicates. Some other times, alumina generates its own 3-D structures alternating with those made of silica tetrahedrons, forming yet another type of silicate mineral featuring alternating silicon- and aluminum-based sheets; that’s why we call them layered silicates or “phyllosilicates”. Micas show this type of sheet structure to the naked eye. Clay minerals have the same structure but the crystals are micron-sized. Some asbestos are also formed by phyllosilicate minerals like “serpentine”: the layers are folded on themselves forming the fibers we see with the naked eye.
What kind of mineral forms depends on what is available at the moment in that place. And usually it all begins in a magma. Magma is just molten rock. Or we can see it the other way around: when a magma cools down it solidifies into a rock - rocks are solidified magma. A planet is made of rock.
When the Earth formed it was a ball of magma, molten material from the condensation of a rotating dust cloud around the forming Sun. Temperatures and energy were so high that the only state possible was the liquid one: molten magma. As the magma cooled down, under certain temperatures some of it at the surface became solid. When this happens, the molecules join together to form oriented structures that can grow to visible crystals - the minerals. Which kind of minerals depends on the magma composition and on temperature and pressure conditions in the place where the cooling is happening. Since we defined magma as molten rock, we can think of magma as a mix of silicon tetrahedrons with iron and alkali atoms waiting for the temperature to drop to the point they can bond with them. Silicon availability mostly dictates what kind of minerals will form as a magma cools down.
Magmas rich in silica will generate a variety of silicates containing both metals and alkali. When the silicon is scarce, it will be easier for the magma to generate silicates with fewer tetrahedrons bonding more metals than alkali. In this case the resulting rock will be referred to as “ferromagnesian”, having a higher content of metals, namely iron and magnesium. There can also be some alkali there, but sodium, potassium and calcium are more widespread where silicon is abundant. Here, silica in excess will be free to crystallize as quartz. This kind of magmas/rocks are called “oversaturated”, referring to silica content being so high that when all other minerals are formed there is still some to generate quartz. Ferromagnesian magmas and rocks are typically undersaturated in silica: there is merely enough to form some olivine and pyroxenes.
They are also called “mafic”, having a higher content of magnesium and iron (ferrum in Latin). On the other hand, “oversaturated” alkaline magmas and rocks are called “felsic” (containing feldspars). An older way to define a magma/rock type was to call “basic” those with fewer silicon concentration and “acid” those rich in silica. That’s because it was once believed that silicate minerals were the result of the reaction of a kind of silicic acid with metals and alkali. There is no such an acid but the nomenclature stuck for a while and I’m still used to it.
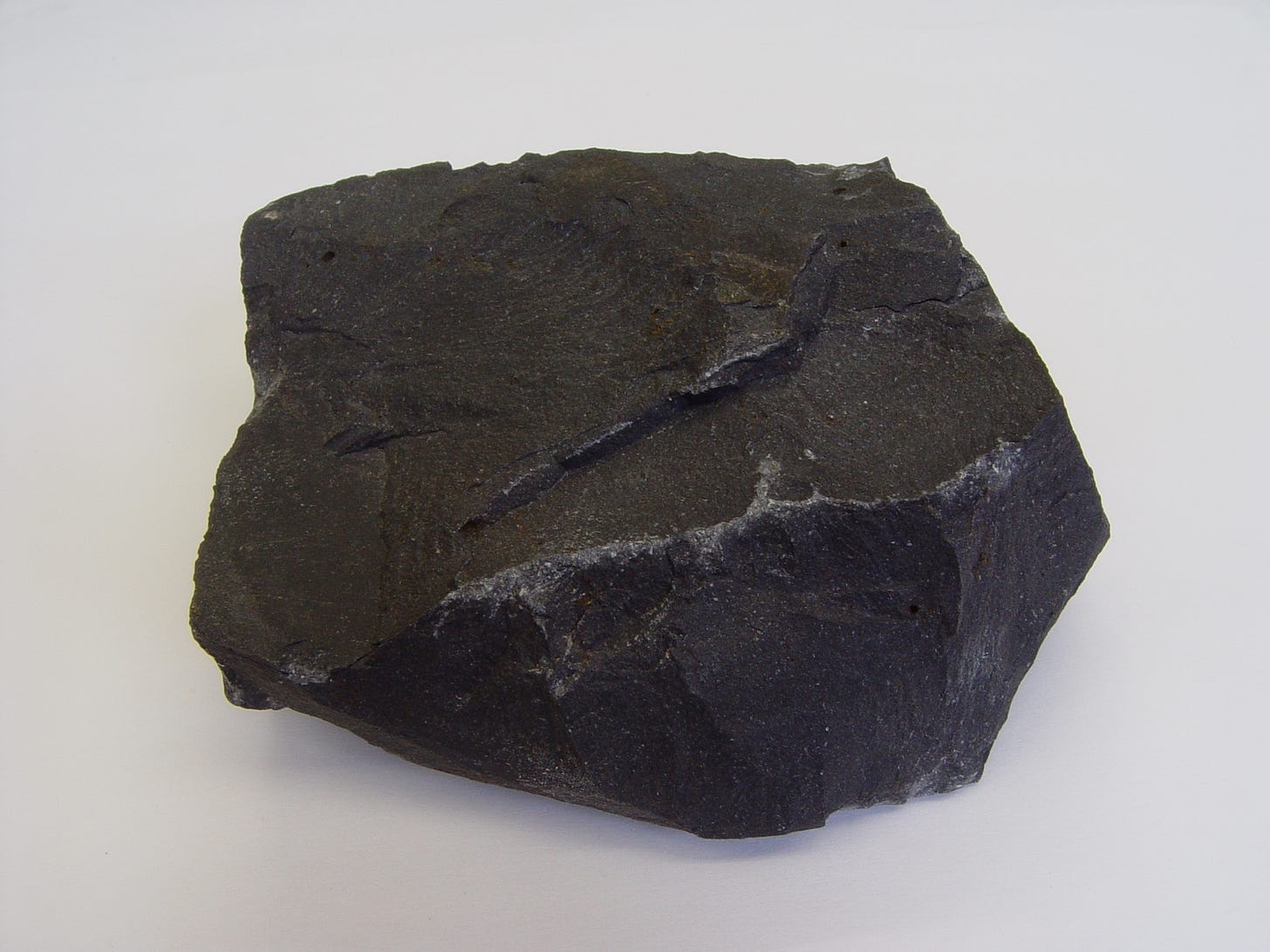
Typically, a “basic” magma, undersaturated in silica, will solidify in rocks we call “basalts”. Most lava flows generate basalt. The relative scarcity of silica tetrahedrons makes the magma more “fluid”. The lack of dissolved water and alkali makes its solidification temperature higher (1000-1200 °C), helping the magma flow easily as lava. On the contrary, more “acidic” magmas, richer in silica, are more viscous because of the higher friction among the abundant tetrahedrons. This also allows this kind of magma to trap dissolved volatile gases such and water vapor more easily; therefore, the magma exerts higher pressure against the confining rocks and is more prone to explosions. High-silica content makes it more difficult for a magma to reach the surface (and when it does it is often devastating). That’s why most of those magmas slowly solidify underground when they cool down below about 500 °C, forming intrusions within the confining rocks. Those are called “plutons” or “dykes” depending on their massive or layered shape. The most common rock forming those intrusions is called “granite”.
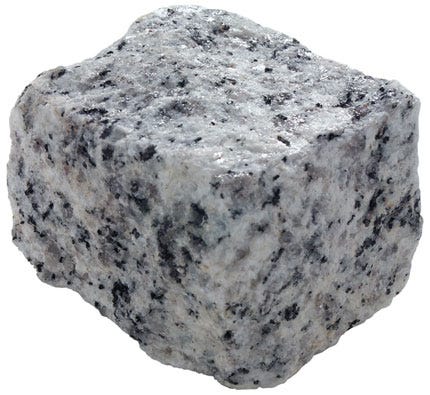
Therefore, undersaturated magmas are prone to generate effusive eruptions to form basalts; if they are trapped underground, the intrusive rock is called “gabbro”. Oversaturated magmas are prone to generate intrusions called granites; otherwise they may cause explosive eruptions or viscous lava flows forming a rock called “rhyolite”. Basalts and gabbroes have the same composition, they are made of the same minerals. The minerals’ grain size is different because they formed in different conditions. Minerals in lava flows form rapidly as the magma cools in the air or water: the crystals have no time to grow but they’re still there, although you need a microscope to see them. The same goes for granites and rhyolites: same composition but different grain size. In a granite, that slowly crystallized underground, you can spot quartz as the translucent crystals or felspars as the whitish ones.
Of course, these are the two extremes. Between them there are a variety of intermediate compositions like andesites (less undersaturated basalt) or granodiorites (less saturated granite), often because a basic magma can differentiate itself towards more acidic compositions during its evolution. This are the rocks we call “igneous” or “magmatic”, since they are generated from cooling magmas. All those rocks, when exposed, undergo degradation by weathering. Erosion will alter them into differently sized particles or “clasts”; they will be transported by winds and water streams to eventually deposit as sediments and later become sedimentary rocks like sandstones, siltstones and shales (by decreasing clast size). Rocks can be buried so deep or compressed in tectonic movements that they alter into other kinds of rocks known as metamorphic rocks. Temperatures and pressures can be so high that rocks can melt into a magma and the cycle starts all over again. Ferromagnesian rocks are more widespread in oceanic crust, while felsic rocks are the average composition of the continental crust. But that’s another story…