I often notice that even other well-trained scientists when talking about geology sometimes make trivial mistakes. Perhaps, because Geology is a relatively new science, it is little known. for example, its other "cousin" science (because it is also a "historical" science) Astronomy, is far better known. Probably looking up, toward the stars, makes us dream more than looking down, underground. Furthermore many associate the geologist with her or his freelance profession, the one related to construction, surveys, and the study of the technical characteristics of soils. But that is only one of the many applications of Geology. Others may be the search for subsurface resources, the study of geological risk (earthquakes, eruptions, landslides, floods-themes that perhaps explain the greater fascination of Astronomy than Geology). I could go on but I want to dwell here on what is really typical of the geologist, whatever activity he or she does: the geologist's field work.
What does a geologist do on the field? Geological surveying. I am told that this typical geologist's skill since a few decades ago is becoming increasingly rare. Many young and old geologists seem to prefer to work on the computer, which is very useful in so many aspects, I'm fully aware of it. I am just pointing out that the number of students choosing to do a thesis that involves more field work has dropped tremendously. I may be old-fashioned by now, but whatever a geologist is going to do, if he or she has not gone through a good training in field geology he or she will be missing something. That something that really makes the geologist unique. I'll try to tell you about it.
Let's get back from where I emphasize that Geology is a science, one of the Earth Sciences. Its technical application of which many make a profession that requires registration with the National Order, is one of the various facets. Geology studies our planet, the Earth, its evolution, dynamics, structure, etc. The various branches (volcanology, geophysics, paleontology, mineralogy, sedimentology, hydrogeology, etc.) can be very specialized and some are in common with other sciences (planetology, geophysics, etc.). I was saying that Astronomy is the other historical science, which has to do with the passage of time; both deal with a very distant past, measured in millions if not billions of years. The two sciences are cousins because the Earth is a planet; planets are celestial bodies, the object of study of Astronomy. Planetology is concerned with the study of planets, inspired strongly by the knowledge we have of our own, hence by Geology. Planetology can thus be seen as a branch of both Geology and Astronomy.
But in the end the geologist is seen as the "weird one" who likes rocks. What is so fascinating about rocks? Even in school, rock classification appears to be one of the most boring subjects. Geology in schools, in my country at least, is taught little and often very superficially. Unless the teacher causally has a degree in geology, he or she is often not prepared enough to make its study interesting. And then the school programs are deficient as far as the geology part is concerned; it is too little considered. This is probably a major cause of the trivial mistakes other scientists make when they talk about it, as I said at the beginning (not to mention the mistakes public administrators make when I have to make decisions about it!).
But let us get back, then, to rocks. They are defined as aggregates of minerals, which are nothing more than crystallized compounds. They fascinate the geologist first of all because a planet is made of rocks. One has to know them to understand them (thus know mineralogy and petrology). To work on the field, it is crucial to know the rocks of the Earth's crust, the outermost film, some 50 kilometers thick at most. The most important ones are formed by silicates of iron and magnesium, as well as silicates of alkali minerals (calcium, sodium, and potassium), Those in the crust are the only rocks we have touched. Those in the mantle and core we can only speculate on. A rock tells a story to a geologist who can read it. A succession of rocks, with the oldest at the bottom and the newest at the top, is an open book because each of them formed in a different environment that changed during geologic time: different rocks form in different environments. This is most true for sedimentary rocks, one of the three major groups along with igneous and metamorphic rocks. Simply put, igneous (or magmatic) rocks are those that result directly from the solidification of magmas (magma is simply molten rock). Erosion dismantles them into sediments that once buried become sedimentary rocks. Under higher and deeper pressures and temperatures, the minerals that make them up are transformed and the rock becomes metamorphic. The study of all these steps is feasible by observing them in nature, in geological succesions. The fascination lies precisely in reconstructing the processes that led to the formation of a certain geological succession. The point is that geological successions are rarely easily observed (such as in the Grand Canyon). More often than not, a piece of it pops up here and there, among the vegeation, in roadside ditches, quarries, or on slopes devoid of vegetation. And this is where the skill of the field geologist comes in: from partial observations we are able to reconstruct the puzzle.
The geologist's weapons in the field are characteristic: the hammer with which to take rock samples, the lens for analyzing them, the magnetic compass for orienting oneself but above all for taking the measurement of geological surfaces to put on the map, along with color pencils to map the outcrops of different rocks observed. And the field notebook where to jot down everything: it's invaluable, must be durable but pocket-sized, and must be defended with your life, because it contains all the work. Note that it is advisable to use a pencil to write on the notebook: in case of rain or recovery from a stream (it happens), the ink would be washed away, the pencil stroke would not (not to mention that it is easier to correct it). The field geologist will need to be able to walk even difficult, steep trails, perhaps even along ravines or escarpments, cross streams if necessary, calculate the time back to the car or base before dark. Proper boots are needed, protection from rain and/or heat, humidity, wind, and perhaps from unpleasant encounters: sheepdogs are a minimum, poisonous snakes and bears perhaps would be good to avoid. If the weather forecast is not favorable it is a good idea to postpone.
Today maybe you do everything with an internet-connected tablet with internal GPS and appropriate software but under rain these tools can have limitations if not specially designed. However, the "craft" still needs to be known: there is not always internet coverage and batteries have limited life.
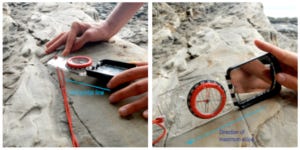
We usually begin by analyzing maps, the topography of the area to be investigated. The distribution of water streams, their network already suggests the main structures, highlighting areas of major or minor erosion. Obviously a careful reading of all previous geological work and the acquisition of the official geological map of the area are vital. Analysis of aerial photos, nowadays somewhat feasible even through Google Earth, is equally very useful. The use of computers makes it possible to recreate 3-D models of the terrain that help in a preliminary interpretation of the morphology, even draping it with satellite imagery. It will also possible to drape it with our geologic terrain map to verify the validity of our three-dimensional assumptions in the field.
The landscape suggests geological structures; one must know how to interpret it. The first step is usually an inspection by car, to be planned on a map, first on the main roads, then on secondary roads. In addition to an initial study of the landscape, it is useful to search for major outcrops, road cuts or any other point where the bedrock, that of the subsoil, not covered by the product of its alteration, the soil, is laid bare. It will thus be possible to optimize the routes to follow to analyze each outcrop. But these will be the easiest to reach. We will then have to plan to walk the available tracks, always looking for other outcrops to analyze, not forgetting to reason from the most scenic points about what the morphology suggests (trivial example just to understand: more erodible rocks era in the valleys and more resistant rocks in the peaks).
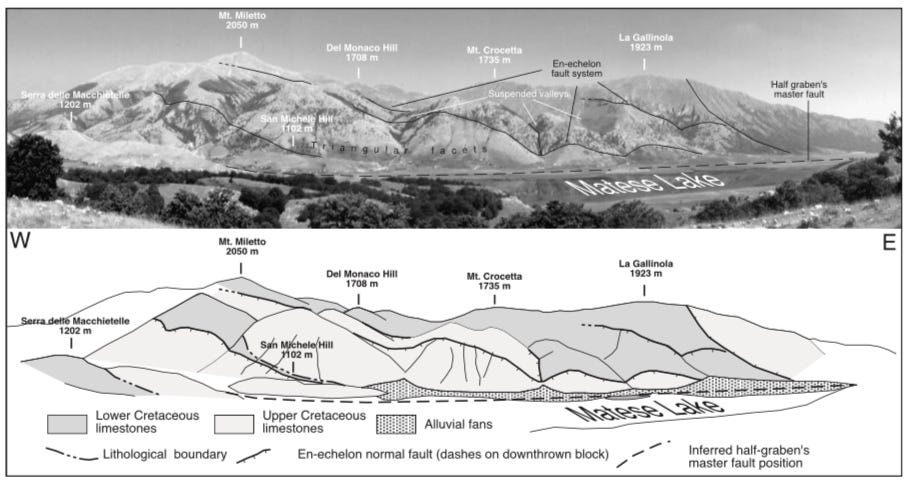
But what do you do once on the outcrop? You have to recognize the type(s) of rock exposed. Unless you are in a protected natural park, you will use the hammer to take a sample of it and also examine it with a 10x magnifying lens. In order to observe rock characteristics, you need a fresh cut. The surface is altered by exogenous agents, it can still suggest something, but to see the mineralogical and paleontological content, you need a fresh cut and the magnifying lens. Having taken the rock sample, the geologist ...licks it. Oh yes, it is really love between the geologist and the rock! No, especially on limestone rocks, if the surface of the fresh cut is not wet the magnifying lens does not help much! Sure you can pour some water on it (but it is precious for our trek), you could spit on it but then you have to smear the saliva with your finger, making it dirty. Eventually one resigns and learns the classic, quick fix method: give it a good lick and investigate with the lens. After all, it's not like you lick the altered surface of the rock, who knows what bacteria, mosses, lichens, etc. are there? Fresh breakage exposes something that may have been there for millions of years, unless you know that you are likely to encounter rocks rich in arsenic or other toxic elements, rest assured that no organisms may have survived. Split, lick and observe: far too convenient, it becomes a normal thing after a while. In addition to figuring out the grain size of minerals that make it up, the eventual fossiliferous content also allows the experienced geologist to infer the age of the lithology she or he is observing. One is looking for any fossils that indicate a particular time in the Earth's geologic history. In sequences of marine origin (sediments deposited in the sea, then turned to rock and brought back up by the formation of mountains) one looks for clues to the content of planktonic fossils: their small shells are sometimes visible with a magnifying lens, and the experienced eye can date a rock directly in the field if he or she recognizes a fossil marker! These are species that evolved and became extinct within a few million years: if spotted right on the field they give a big help in geological reconstruction! Otherwise, one can collect important or doubtful samples to be later analyzed, or have an expert micropaleontologist analyze them, in the lab under a microscope.
There can be different rock types in one outcrop, and they should all be analyzed and described on the precious field notebook, on which there will be a proper label, which should also be placed on the map, at the exact location of the outcrop. Colors will also be used to mark the outcrop on the map, different colors for different lithologies. Another important thing are the observable geological structures, first of all, the bedding if any.
Bedding is paramount. Often rock come layered in strata. That layering is to be measured with a geological compass, which unlike any other compass, also has a clinometer, a pendulum or similar system for measuring the inclination of lines (plunge) or surfaces (dip) with respect to the horizontal: a horizontal layer has a 0° dip angle, a vertical one 90° dip. With the compass needle, we measure the direction, or "strike" angle, of the layer with respect to the north: a surface is defined by infinite straight lines; if we think of all the infinite horizontal lines that make up a sloping surface, they are obviously all parallel to each other and identify a direction with respect to north. By placing the compass in the direction of these straight lines, parallel to them - that is, in the direction of the layer or other plane - we can measure the angle between the north-aligned needle and the compass, which is aligned with the geological surface. This will give us its strike angle, for example N-30°E. It means that the direction of the surface is obtained by by turning 30° moving from the north to the east. To this measurement we must add the dip angle of the layer, to be measured with the clinometer of the geological compass. Another method is to take the dip's direction altogether. The important thing is to specify your method clearly in the report and, of course, to record all measurement on paper with the appropriate symbol: a segment oriented as the direction of the stratum joined to a shorter one drawn perpendicularly from the middle of the first one with the dip expressed in degrees.
Correctly measuring the strike and dip of the strata in the various outcrops will give us insight into the relationships between the various lithologies observed (simply put, which ones are above and which ones are below). The basic rule is that the oldest rocks are below the newest ones. But in mountain ranges it can happen that the strata are deformed and folded to such an extent that we might happen to be observing an inverted limb of a fold: the older strata would be on top and the younger ones below. A skilled geologist should figure it out!
The layers of an outcrop may be visibly folded. It should all be reported on the notebook thoroughly, with the aid of a drawing on which to record notes and measurements (yes, a geologist should also be good at freehand drawing). It is useful to take photos that can then be linked back to the correct outcrop described in the notebook. There are smartphone phone apps that allow us to take geological measurements without a compass (by orienting the phone as the bedding); the phone also takes the location on the map and reports on it all the measurements of strata and various structures with the appropriate symbology. Needless to say, we can take photos (which are georeferenced on the same map) on which to draw patterns and highlight notable features of the outcrop.
For example, it may happen that we observe two different lithologies in two different places and we know that one is older than the other but that, from the arrangement of their layers, they appear to be at the same level (while the older one should be lower). Often this means that a fault has displaced the geologic sequence by moving two blocks over time (millions of years) bringing the older rocks to the level of the younger ones if not even above. Sometimes faults are not visible but are interpreted with these kinds of field observations. When you are luckier faults crossing displaced strata are observable in the outcrop. The direction and slope of the fault plane(s) should be measured, reported in the booklet and map, describing their behavior (how far and in what way each appears to have displaced the strata).
The ultimate goal is to identify and map geological boundaries. faults for example are tectonic geological boundaries. The geological boundaries that we aim to identify and/or interpret in the field are the lithological boundaries. Roughly speaking, since this is not a field geology course, these are surfaces that delimit rocks, or groups of different rocks, i.e., geologic "formations" that are well defined as lithology (type of rock or rocks), fossiliferous content (hence age), lower boundary (bed) and upper boundary (top). Lithologic boundaries are surfaces ( plane or deformed) oriented in space; during geologic surveying we can measure their orientation (strike and dip) with a compass at different points in the area. Geological mapping's specific geometric procedures (stratimetry) allow the reconstruction of the intersection of lithological boundaries (and any fault planes that displace them) with contour lines so that they can then be mapped. Thus a geological map is born.
Day after day we will have accumulated data to think about, to put together to reconstruct the geology of the area we are investigating. It is fascinating work that requires, in addition to knowledge of the subject, good three-dimensional visualization skills and an investigative intuition similar to that of a detective if not a ... physician. My geology and surveying professor used to say that it is like being a great expert on cars: by looking at a small part, a handle, a headlight, you can trace the make, the model, maybe even the period of manufacture, the age. Different types of rocks were formed in specific environments. Reconstructing their succession, the relationships between them, is equivalent to reconstructing the past of an area, how the environments evolved there over geological time. It is a journey back in time, millions, even hundreds of millions of years. The Earth is about 4.5 billion years old. Rocks that old are no longer there, but keep in mind that a few million-year-old rock can also be seen as recent!
When a geologist looks at a rock sample, he or she is aware that it was formed in that place millions of years ago. It contains information about its history and that of the area in which it was found that only a geologist can appreciate. Mine is an attempt to communicate the fascination of a science that is actually little known and understood by the uninitiated. Not only out of respect due to the professionals and scientists who pursue it, but also because knowing about geology helps us to make better decisions when choices have to be made that affect the environment around us.
Somewhere I read that to most people mountains appear still and immense, only a geologist perceives their fragility and motion.