Geologica 2 - Moho's mojo
How the coolest Croatian geophysicist and meteorologist discovered the crust-mantle boundary
Andrija Mohorovicic was a brilliant child. He was born on 23 January 1857 in Volosko, a small fishermen village in the Adriatic coast of Croatia, then part of the Austrian-Hungarian Empire. His father was a blacksmith making anchors for ships. Andrija inherited from him a love for the sea. Andija’s mother died at 42, when he was only 6. His father married again and had 7 children from his second wife. Andrija’s stepmother had always been kind to him, too. While at elementary school, a priest who was also a teacher quickly noticed that little Andrija was extremely gifted, so he persuaded his father to send the boy to continue his studies and he enrolled in a high school in the nearby Fiume. By the age of 15, Andrija was able to speak English, French and Italian.
After school he attended Prague’s University and learned also German, Latin and Ancient Greek. He earned his mathematics and physics degree in 1879. Back home he started teaching at schools. He was appointed by the Royal Naval School to teach meteorology and oceanography. Later he moved to teach at the Main Technical School in Zagreb where he was named director of the meteorological observatory. He received his Doctoral degree from the University of Zagreb in 1897 and became university professor, teaching geophysics and astronomy.
He was a heavy smoker and a gentle and reserved man. He seldom went out in the evenings and after the loss of his son Francis he became even more private. Since he was a high school teacher he conducted studies of the atmosphere (teachers of the time had also research duties). He succeeded in having the climate control center moved from Budapest to Zagreb and also established the use of the the exact time in his country.
On the 8 October 1909 there was a strong earthquake in central Croatia, near Pokuplje in the Kupa Valley (40 km south of Zagreb). Thanks also to him, for the first time there were several seismographs that recorded the earthquake and there were a lot of data available. Andrija was examining the seismographs from the various sources at different distances from the epicentral area (identified as the place where the earthquake caused the major effects).
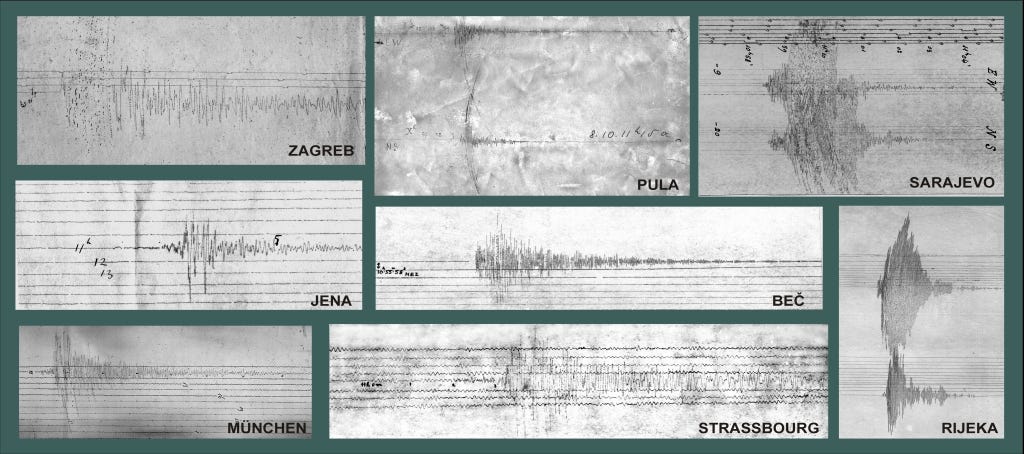
Earthquakes generate two main different seismic waves, the compressional P waves and the transverse S waves. The latter are slower and the delay from the P waves increases proportionally with the distance form the measuring stations. So Andrija plotted the S-waves arrival times versus the distance from the epicenter: the ratio between space and time is speed - this would allow him to estimate the seismic waves velocities. He was expecting to be able to fit the dots (distance/arrival-times couples) with a straight line whose angle with the distance axis would be the inverse of the wave’s velocity (1/V). But a straight line would not fit the dots in the graph. The farthest recordings would be fitted with a slightly less steep segment, indicated a higher speed: the seismographs from more distant stations recorded higher seismic waves velocities.
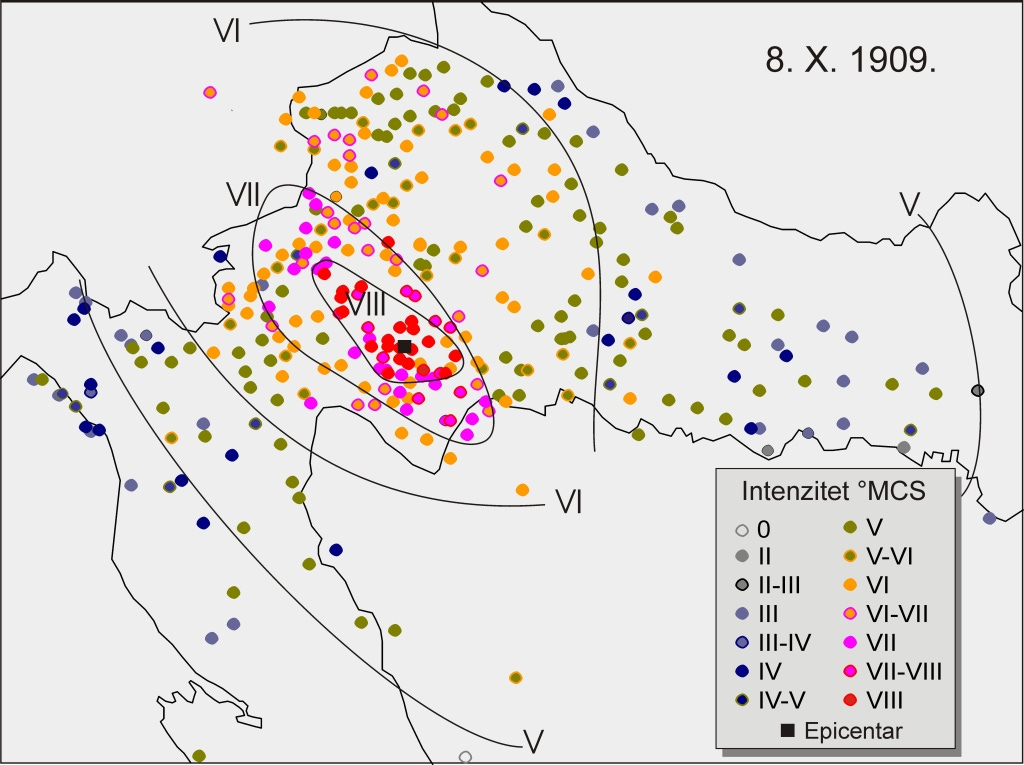
The velocity of a seismic wave depends on the properties of the medium it is traveling in. For the sake of simplicity we can say that the higher the density, the higher the speed1. It was known at the time that the Earth’s density was likely to increase with depth, so a speed increase could also be expected. Andrija concluded that seismic waves had passed through different a material of different composition from the one where the earthquake had originated. This different composition must have caused the seismic waves to increase their speed.
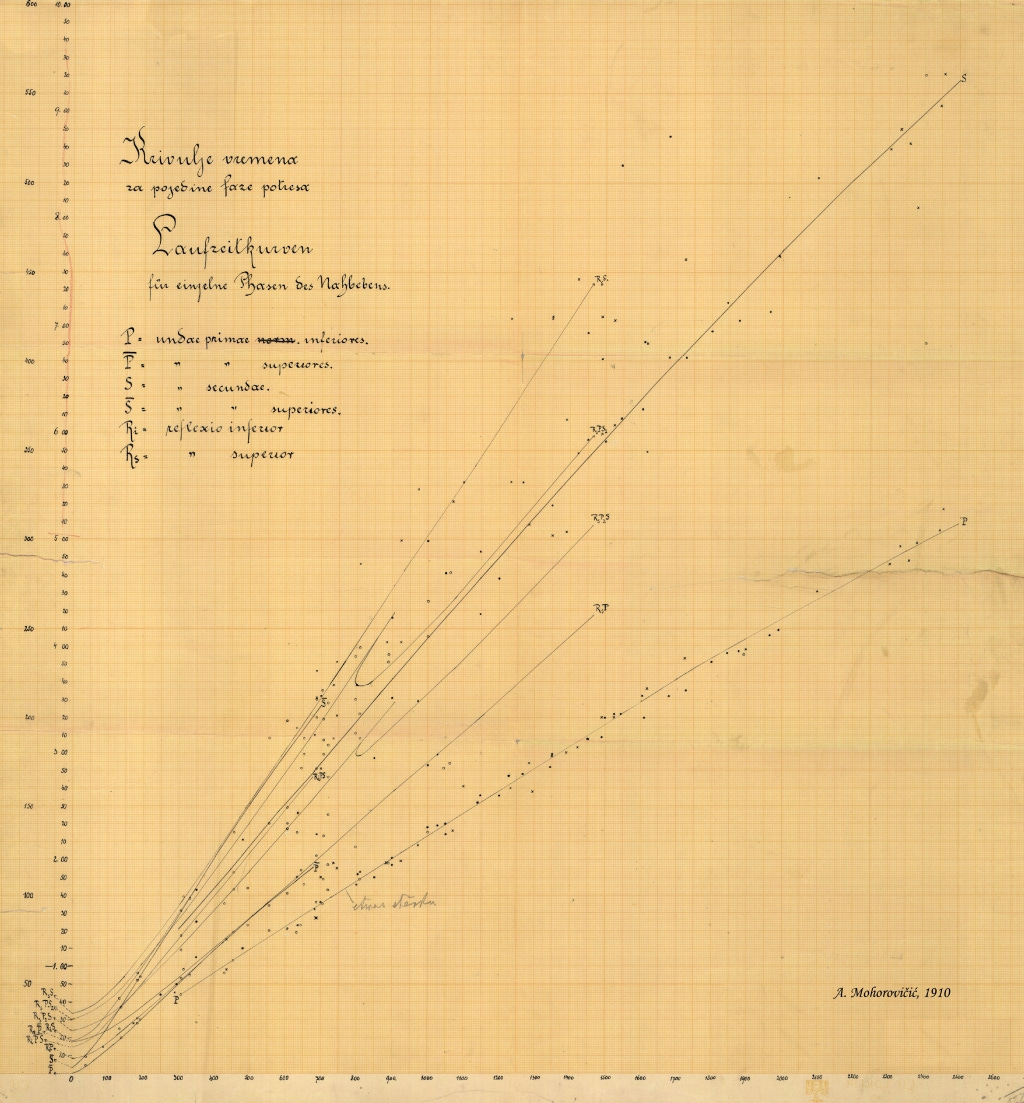
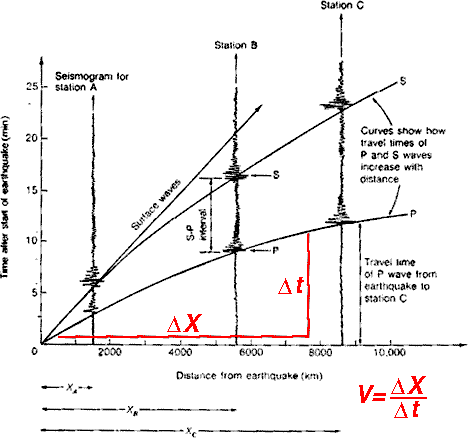
When a wave intercepts a boundary between two layers of different density, it is partially reflected (bouncing back away from the boundary surface) and partially refracted (passing through the boundary but deviating by an angle that is larger for bigger density differences). For instance, if a seismic wave traveling downwards intercepts a boundary with a denser rock layer, some of its energy will be reflected back at the same angle from the vertical at which it met the boundary; some of the energy will pass the boundary but, being the material denser, it will accelerate to a higher speed and be deviated to a larger angle to the vertical. That is a refracted seismic wave.
In simpler words this means that refracted seismic waves don’t have straight raypaths within the Earth: the continuing refractions as they go deeper through denser and denser layers generate curved raypaths that will eventually bring the refracted waves back to the surface at a certain distance form the source. Waves traveling vertically downwards cross the entire planet and theoretically emerge at the antipodes. All other raypaths starting at any other direction than vertical will be continuously refracted away from the vertical until they come back to the surface: the closer they start to the vertical, the farther and deeper they will travel.
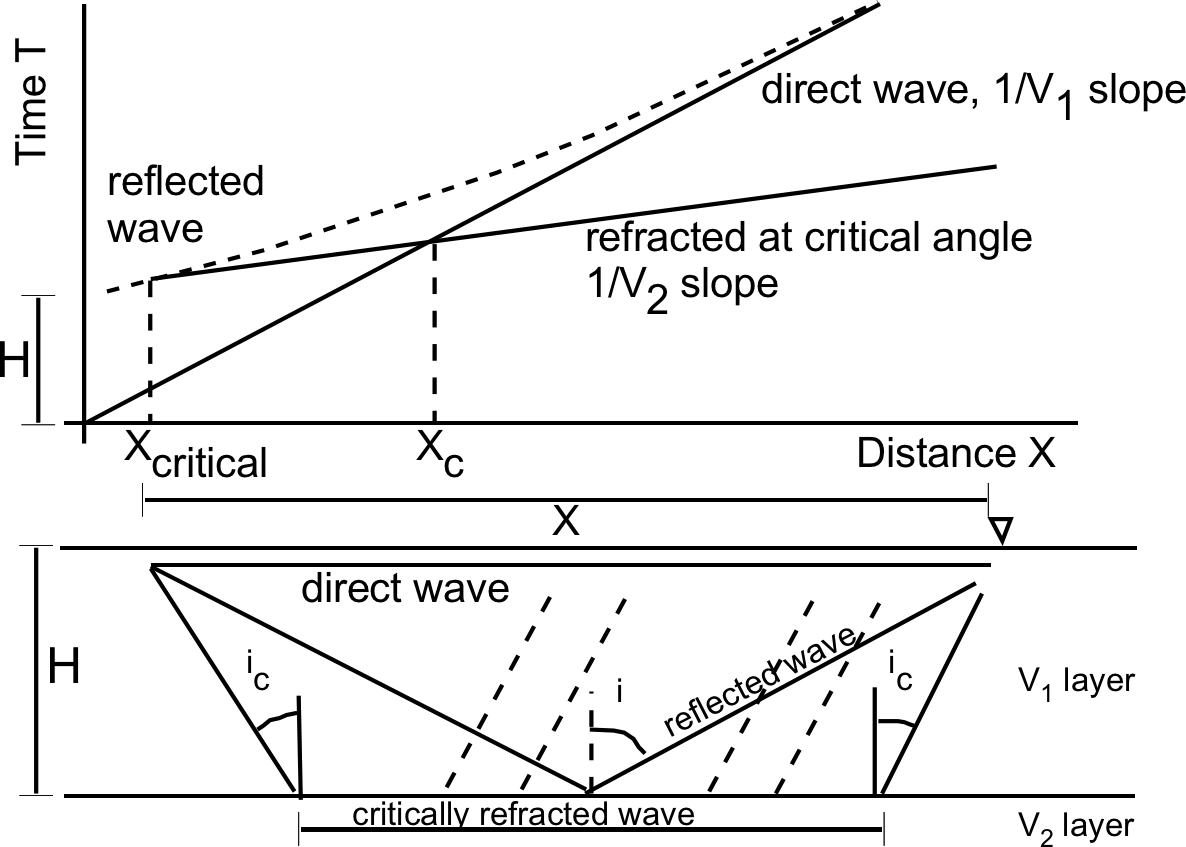
As a seismic wave is continuously refracted by successive deeper slightly higher speed layers, we can imagine a certain depth at which the refraction angle will deviate the wave along the boundary itself. This is called the critical angle. The boundary will become the source of refracted waves all emerging from it at the same angle as the critical one (picture above).
These knowledge allowed Andrija to calculate the depth of the layer with different composition under the area of the earthquake. The boundary surface or discontinuity separates the Earth’s crust above from the mantle below. The surface bears the name of Mohorovicic discontinuity or simply “the Moho”. The change of composition occurring at the Moho causes a speed jump from about 6 km/s to nearly 8 km/s. The crust thickness Mohorovicic calculated was around 50 km. The Moho around the world ranges between 25 and 60 km in continental areas (up to 70 km under mountain belts), while it is below 10 km under the oceans.
From surface and well data we know the average composition of the continental crust as being close to that of granite-diorite rocks (rich in quartz, sodium silicates, and calcium silicates); the oceanic crust is poor in quartz and its composition is close to that of basalts (rich in iron and magnesium silicates).
For what concerns the mantle, rocks from deep volcanic conduits suggest a peridotitic composition (iron and magnesium silicates with low silica content). By studying the properties of the seismic waves that we know have crossed the mantle we can derive crucial information about its characteristics. With principles similar to those that helped Mohorovicic identifying its upper limit (the Moho), scientist have been able to locate at least two major changes in the speed of seismic waves and define two discontinuities within it at 410 km and 660 km depth. We therefore have a 3-layer mantle, the upper mantle, a transition zone, and the lower mantle.
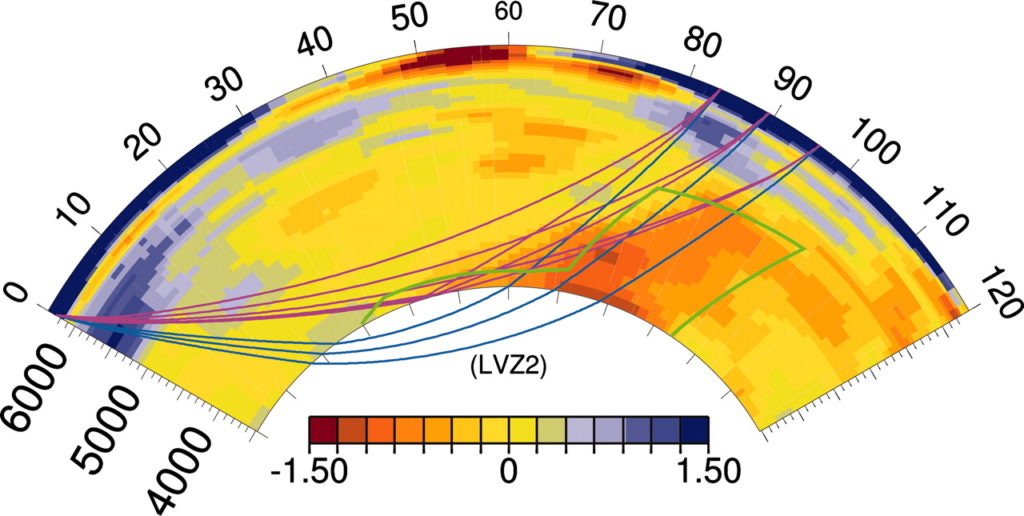
The upper part of the mantle contains an area of low seismic velocity that we call the Astenosphere. The low seismic velocity (and an almost cancellation of the S waves) must be due to areas partially melted in the upper mantle that would allow isostatic movements and as we will see later, those of the plates. Basically these are materials in a state similar to that of a toothpaste that reacts rigidly to rapid impulses such as seismic waves, and fluidly to slow impulses such as tectonic movements.
The transition zone (410-660 km) is due to the abrupt thickening of the minerals that compose it. The crystalline structures adapt to the enormous pressures that occur at these depths, generating structures typical of the mineral called spinel.
The lower mantle is much stiffer than the rest of the mantle, showing characteristics similar to perovskite-like crystalline structures. Although the temperature is very high, the pressures are such that the rocks are not very ductile. Obviously it is the least known and most debated layer of the mantle by geologists and geophysicists.
As of today, no one has ever put their hands on the mantle directly, no borehole has ever reached it. What we know about it is from indirect data (like seismic velocities) and a lot has yet to be done to understand its properties and features. Andrija Mohorovicic has been among the first to tap into this enigmatic Earth layer (which makes for almost half of our planet’s volume - the crust is really thin compared to it). As scientists still do today, he used indirect geophysical methods and was able, in the early 20th century, to locate the top of the mantle. “The Moho” is the name we all use affectionately in honor of the discoverer of this mysterious and fascinating boundary.
For the more curious: in the seismic wave velocity formula, density is at the denominator! At the numerator we find elastic moduli of the material: they increase so rapidly with density that they determine the higher velocity for denser materials.